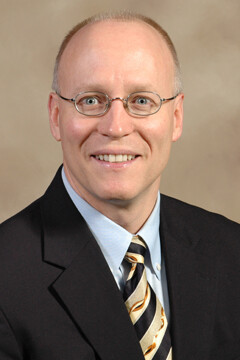
The unusual collaboration between UNL engineers and Purdue University plant biologists has helped those researchers gain a better understanding of how plant cells grow and how they gain their unique shapes.
Two UNL mechanical and materials engineers — professor Joe Turner and postdoctoral research associate Anastasia Desyatova — partnered with Purdue agronomy professor Dan Szymanski’s team as it studied trichomes, the hair-like structures on leaves. The findings from the project, a two-year study funded by the National Science Foundation, were published in the March 2 issue of Nature: Plants.
Szymanski said the use of computational modeling took this project down a unique path of knowledge discovery. The predictions generated by the modeling led to unexpected and fruitful paths of experimentation that otherwise might not have happened.
“You could sit around and discuss this and concoct many different possible explanations, but what Joe and Anastasia did was really powerful,” Szymanski said. “They looked at over a dozen different parameters and identified a few that have a strong effect on shape change. We were able to test those predictions.
“I use the term synergy,” Szymanski said. “By combining the experimental biology and the predictive modeling we were able to learn a lot about how wall texture is controlled and what parameters of the wall really matter for cell shape control – parameters like wall thickness gradients and zones of isotropic character – wouldn’t have been predicted.”
The project studied the development of trichomes – small hair-like growths – on the leaves of the Arabidopsis thaliana plant, a small, flowering Eurasian mustard green. While it was well-known that the trichomes keep insects and other things off the leaves, little was known about how and why the plant and its individual cells take their unique shapes.
Turner and Desyatova used computational modeling to help understand the process, and some of the questions that arose from the modeling led to new areas of research within the project. Some of what the researchers learned, Turner said, was not what they logically would have expected.
“The thought was that the easiest way for a cell to grow would be to have a sphere ballooning outward. That’s not how this cell grows. It stretches out in a very specific direction and at the end that has a tip that isn’t perfectly spherical. It changes as it grows,” Turner said. “Our work was focused on the development of a computational model of the growth process and how the cell changes its shape during growth.”
One of the areas that puzzled researchers was how protein complexes pattern the depositional material on cell walls. Szymanski’s team at Purdue had done optical measurements of the cells and Turner’s team created realistic computational models to provide explanations. One finding from the model was that the thickness of the cell was integral to the change in shape during growth.
“We had this nice back and forth between the measurements and the modeling. We tried to model their growth results (the cell shape) with our model, but we couldn’t get good agreement unless we added a thickness variation on the wall,” Turner said. “They never knew if that existed. We suggested this variation had to exist for this shape to be true, and Purdue confirmed the thickness variation existed.”
Desyatova said the results of the study could lead to many advancements in many life sciences fields, specifically in the engineering of any crops that are used by humans.
“We connected the cytoskeletal patterning with the cell mechanics, and understanding that we can learn how to, for example, change the shape of a cell and how it is applied to production of cotton fibers,” Desyatova said. “Affecting the shape of the cotton fibers could make them thicker, longer and stronger, and thus more desirable.”
Szymanski also sees another desirable result of this research — using engineers and their expertise to improve plant quality.
“What we learned provided some of the design principles of those cells so you can think of new ways to engineer cotton fibers to improve their mechanical properties and their value,” Szymanski said. “Traditionally, we’ve done this by breeding and selection. Another approach would be to use molecular biology, gene cloning and regulated gene expression to improve fiber quality in an intentional way. That’s engineering.”